7. The beneficial effects of sports on the body
Compared to the hunter-gatherer and the nomadic pastoralist life form, it was probably a settled lifestyle, beginning with the large-scale introduction of agriculture some 12000 years ago, which caused a great change in the average physical activity of human beings. The second major transition occurred with large-scale urbanization, beginning – depending on the geographical area – at most 200-300 years ago. These changes in lifestyle presumably went together with an average reduction in mobility, and, on an evolutionary time scale, they occurred at a rapid pace. Humans undergo almost no genetic changes over such time ranges, so it can be stated that we are genetically not only capable of a greater average physical load than is associated with our modern way of life, but that we are in fact, “programmed” for it. In the world today every third adult (those over 15 years are already included in this category) on average can be said to be inactive (i.e. his/her activity level does not reach the daily recommended quantity and quality), but in some countries with a developed economy this proportion can reach up to 43 %. The ratio increases with age, and is higher among women. The same data for adolescents between 13–15 years is 80.3 %, that is, four out of every five adolescents are inactive; here too, boys are less affected than girls (Hallal et al. 2012). Several diseases can be linked to inactivity, the manifestation of which is preceded by a syndrome called metabolic syndrome. Metabolic syndrome exists when three of the following symptoms are present:
central obesis,
increased resting blood glucose level (hyperglycaemia),
high blood pressure (hypertension),
high resting blood fatty acid level (hypertriglyceridaemia),
low HDL level (High Density Lipoprotein is a type of protein which carries cholesterol in the blood).
Besides these, insulin resistance and a high predisposition to blood coagulation are typically present. These symptoms increase the risk of the emergence of more serious diseases (type 2 DM, atherosclerosis, non-alcoholic fatty liver disease, tumours, neurodegenerative disorders; Richter, Ruderman 2009).
So it is clear that humans are not made for inactivity. Today, the results of much research support the idea that a movement-rich lifestyle can prevent several diseases, disturbances and health anomalies. In the following, we will sum up the effects induced by endurance and strength training, representing the two extremes of the spectrum of workout types; then we will overview the general beneficial effects of sport, without making any claims to completeness.
7.1. The effects of endurance training and strength training on the body
As we have seen, on the broad palette of training types two extremes can be separated, of which the endurance type aims almost exclusively at increasing performance maintained over a long time-frame, while strength training aims at increasing muscular strength. Several transitional training types exist between the two, and the combination of the two workout types is specifically recommended for restoring the harmonic body functions in obese, inactive elderly individuals (Davidson et al. 2009); consequently, it is also an important component of life-style counselling in the fight against type 2 DM (Colberg et al. 2010). The fundamental difference between the two training extremes is that endurance (aerobic) exercise does more to improve cardiovascular parameters, while resistance (anaerobic) training has a greater positive effect on the condition of the musculature and on general ability. The following table gives a detailed comparison of the adaptation responses induced by the two exercise types, and on the effects they have on an individual’s state of health (Table 4).
Table 4: The effects exerted by the endurance and strength training (Egan, Zierath 2013)
- cf. Radák 2016; ** cf. Gibala et al. 2009, Little et al. 2011
7.2. The effects of AMP
Under the effect of physical exercise (or fasting), from the ADP molecules which result from the breakdown of ATP, an enzyme (adenylate kinase) produces ATP and an AMP (adenosine monophosphate) containing one phosphate group (ADP + ADP → ATP + AMP; Figure 19). After this conversion, the AMP cannot be converted into ADP again, but is broken down, and the ammonia (NH3) forming from it will be eliminated through urine.
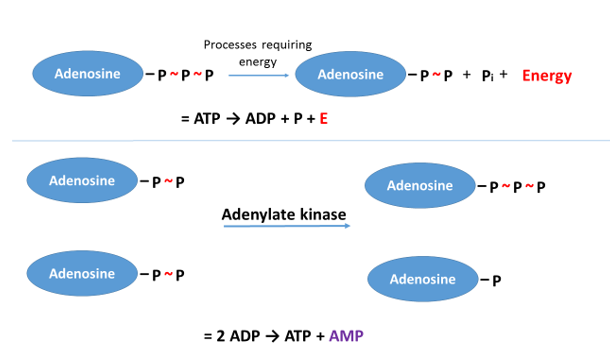
Figure 19: From the ADP molecules, forming from the breakdown of ATP, ATP and AMP are generated
The AMP in the skeletal muscles activates an enzyme called AMPK (AMP-activated protein kinase, which, otherwise, is present in all our cells; Richter, Ruderman 2009) which is capable of binding phosphate groups to other proteins (it phosphorylates them; Egan, Zierath 2013). Phosphorylation is a common “switch” found on proteins which causes them to change their activity, so that the activity of some proteins increases, while that of others decreases due to phosphorylation. The most important triggers of the activation of AMPK are high loading intensity (typically above 60% of VO2max), or a workout of low intensity but long duration (Richter, Ruderman 2009). So the AMPK enzyme, activated in response to exercise, switches a series of proteins “on” or “off”. The level of the AMP, and, consequently, also that of the AMPK in the cells increases proportionally to loading. We will schematically review some important processes which occur under the effect of AMPK.
Since this enzyme tries to conserve and restore the level of ATP, it inhibits the processes of synthesis, and stimulates those of demolition (Egan, Zierath 2013; Viollet 2018). Therefore, it accelerates fat breakdown in the muscles and in the entire body (Richter, Ruderman 2009). Besides this, it inhibits the glycogen- and the protein synthesis of the muscle (Egan, Zierath 2013).
The glucose uptake of the cells increases (Richter, Ruderman 2009). Under the effect of the AMPK, the glucose uptake of the muscles increases, even in the absence of insulin (Egan, Zierath 2013), so in this way, exercise acts against one of the symptoms of type 2 diabetes mellitus, insulin resistance.
The AMPK stimulates the production of a regulator protein called PGC1α (peroxisome proliferator–activated receptor γ [PPARγ] coactivator 1α) (e.g. Handschin, Spiegelman 2008; Kelly 2012), which in turn increases the activity of genes which play a role in endurance; for example, it increases the rate of mitochondrial proliferation (Little et al. 2011, Viollet 2018).
Besides this, PGC1α also raises the rate of synthesis of a protein (fibronectin type III domain containing 5 [FNDC5]) from which a substance which has a hormonal activity, irisin, forms (irisin takes its name from the Greek mythological goddess Iris, messenger of the gods). Under the effect of irisin, the white fat tissue acquires properties which are characteristic of brown fat tissue, that is, it begins to generate heat with great intensity, with which it reduces body weight and improves the quality of glucose homeostasis in the body (Boström et al. 2012, Kelly 2012). Thus, physical activity reduces the degree of insulin resistance in this way as well (Handschin, Spiegelman 2008; Egan, Zierath 2013).
PGC1α lowers the extent of muscle loss in conditions involving a decrease in muscular mass (Handschin, Spiegelman 2008).
Inactivity causes an elevated production of inflammatory factors, so it induces a sort of low intensity inflammation in the body, due to which the likelihood of developing
o certain neurodegenerative diseases (Alzheimer’s disease, Parkinson’s disease, Huntington’s chorea),
o atherosclerosis,
o the already mentioned type 2 DM,
o certain tumours – those of the colon, the breast, the prostate, the endometrium, the pancreas, the skin – increases.
Besides inactivity, obesis also generates, even by itself, a low-grade systemic inflammation, but if inactivity and obesis occur together, then they aggravate each other’s effects. PGC1α, produced as a result of exercise, inhibits the development of these effects. According to estimates, physical activity and a low body mass index together, on average, cause an increase of roughly 10 years in life expectancy (Handschin, Spiegelman 2008).
A positive effect of exercise, especially aerobic exercise, on neural functions is an increase in volume and blood perfusion of the hippocampus which plays a very important role in learning and memory (e.g. Islam et al. 2018), which supports the role of physical activity in improving cognitive functions.
In the bodies of those exercising regularly, the probability values of developing chronic old-age diseases are estimated to be similar to the values which, in the case of inactive, obese people, are likely to occur 20-25 years earlier (Handschin, Spiegelman 2008).
The rise in the level of PGC1α mediating the effects of AMPK, improves the symptoms of depression (Handschin, Spiegelman 2008; Valente-Silva, Ruas 2018).
As an effect of endurance sports, a very interesting adaptation has also recently been described, which can be directly linked to the rate of the ageing of cells. The telomere is a part of the DNA, situated at the end of the chromosome, the size of which diminishes with ageing. Regular endurance and ultra-endurance training reduces the rate of telomere loss, thus, it “keeps the cells young” (Denham et al. 2013, Borghini et al. 2015). The difference in telomere length between ultramarathon runners and healthy controls can be translated into a difference of 16 years of biological years of life (Denham et al. 2013).
The positive effects of exercise are not, of course, mediated only by AMPK, but through the functional complexity of this enzyme we can see how the diminution of energy reserves translates into metabolic changes to be interpreted in the language of the cell. Besides AMPK, several other signal transduction pathways also ensure the coordination of cellular processes and the adaptational steps taking place under the effect of physical exercise. However, the molecules we already know still do not allow us to create a complete picture of the molecular system of training adaptation; this is an intricate and multi-component network, and there are still many things to be discovered about the members, the principal regulator molecules of the network, and the interactions between them. In recent years, for example, it has been pointed out that the – mostly unknown – enzymes activated in response to exercise modulate protein functions in more than 900 phosphorylation sites in the skeletal muscle (Hoffmann et al. 2015).
Thus, the musculature releases several bioactive molecules under the effect of exercise (collectively referred to as myokines) which exert effects on the functioning of very diverse organs: they influence the metabolism of the fatty tissue, the liver, the guts, the pancreas, as well as the metabolism of the muscle itself (Egan, Zierath 2013). This explains why today we should consider the skeletal muscle not only as a passive effector of labour, situated at the ends of the nerves, but also as a large gland, capable of influencing our metabolism to a very significant extent (Egan, Zierath 2013).
The diverse effects of the different types of training, of course, are not manifest to a uniform degree in everyone; however, it seems universally true that sports activity beneficially influences the functioning of our body and psyche, and so is capable of giving us back what we pay to our settled and civilized lifestyle in exchange for comfort.